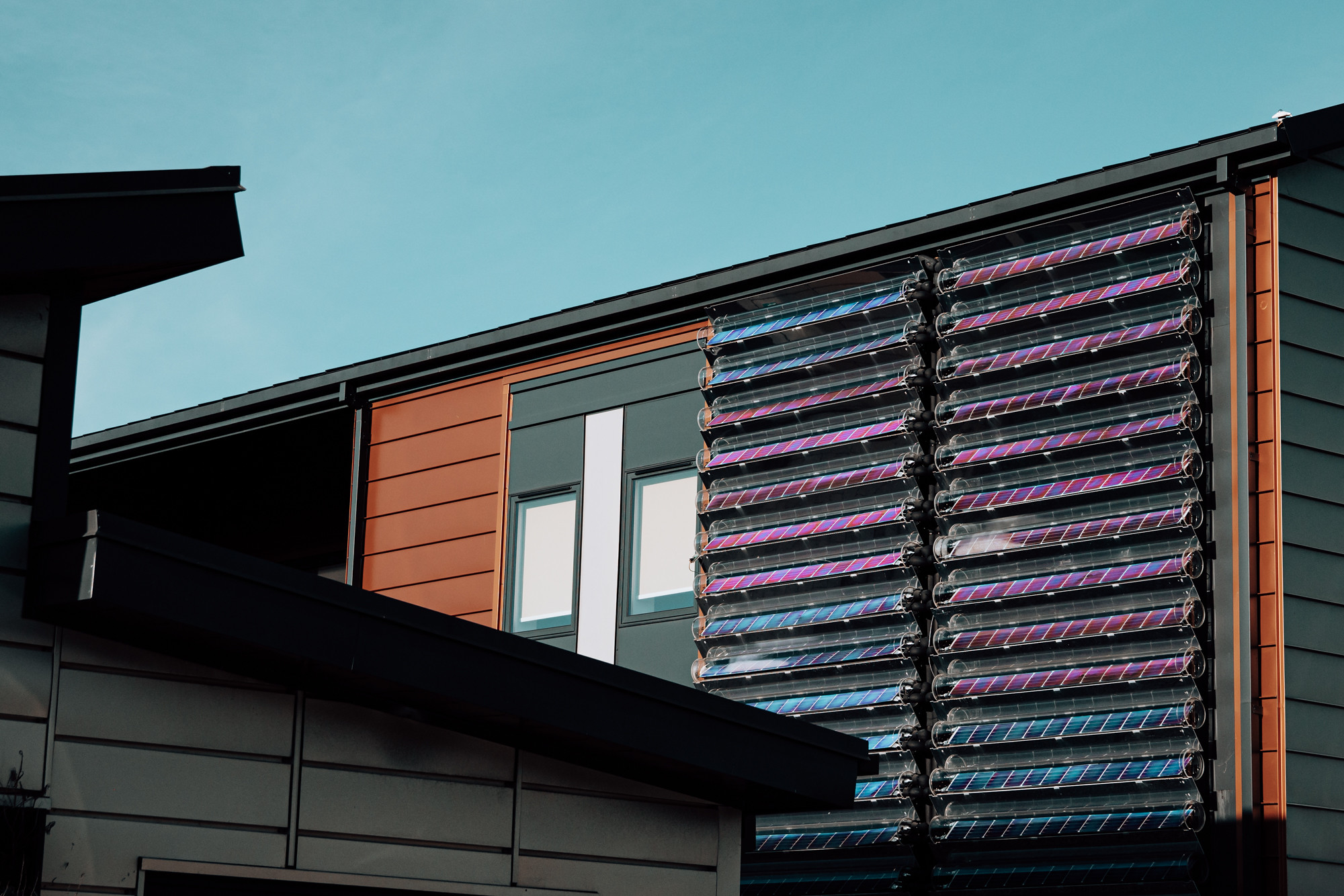
Principle 1: BUILDING FABRIC AND PASSIVE DESIGN
Integrated engineering and architectural design approach including consideration of orientation and massing, fabric efficiency, natural daylight and natural ventilation. Designed for occupant comfort and low energy by following passive design principles.
Design Guide:
- Siting of Building
- Orientation of Building
- Building Fabric
- Glazed Elements
- Natural Light
- Cooling
- Energy
- Water
- Ventilation
- Heating
- Acoustics
- Indoor Air Quality
- Vertical Movement
- Construction Method
- Advice
- Resources
Siting of Building
- If considering use of passive and/or active solar energy, ensure unshaded, south facing facades and/or roofs are available. East and west facing surfaces can also be utilised.
- Undertake early modelling to predict impacts of siting, orientation and correlation with anticipated use profiles
- Consider opportunities for green infrastructure around the building to:
- Reduce flood risk
- Include biodiversity
- Improve health and wellbeing of occupants
- Provide an attractive site
- Improve air quality
- Act as an acoustic or pollution buffer
- Make use of passive cooling and/or shading
- Position within the site to avoid noise or pollution sources. Levels of noise or pollution may influence choice of ventilation system.
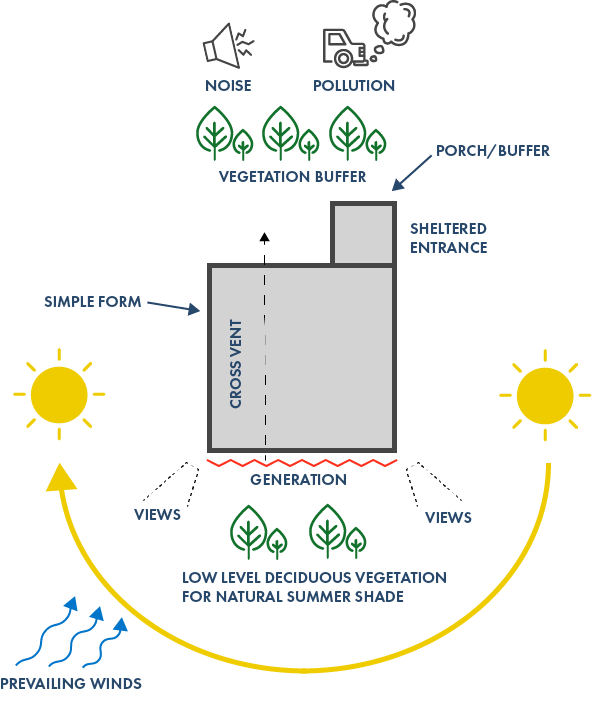
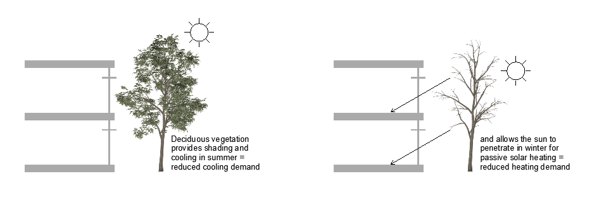
Orientation of Building
- Consider energy generation opportunities (roofs/facades).
- Consider inclusion of green infrastructure, such as living walls.
- Consider ventilation strategy (e.g. if using natural ventilation, openable windows should correspond to the prevailing wind direction – high air pressure on the windward side moves air into the building and low air pressure on the lee face draws air out of the building).
- Consider shelter, e.g. positioning entrance doors away from prevailing winds and/or including lobbies in design
- Consider location of glazed elements
- Consider treatment of glazed elements:
- For natural light
- For passive solar gains
- To avoid overheating and excessive heat loss
- For natural ventilation
- For views
Building Fabric
- Reduce the energy demand of the building through thermally efficient fabric: minimising heat transfer between the exterior and interior of the building, i.e. to reduce thermal loss from the building in winter months (reducing heating requirement) and to reduce thermal gains in summer months (reducing cooling requirements).
- Consider all elements of the building fabric– floors, walls, roofs, windows and other glazed elements such as rooflights, curtain walling, entrance screens, glazed facades
- Renewable energy generation has a better chance of matching the demand profile of a building with a thermally efficient fabric.
- Determine ventilation strategy at an early stage, as this will affect the level of air-tightness required – for use of a mechanically ventilated and heat recovery system (MVHR), for example, an air-permeability of <3 m3/m2h @50pa is recommended, although MVHR can also be effective at lower air-tightness levels.
- Design out thermal bridges as far as possible through a simple building form with minimal junctions.
- Building form factor will affect heating or cooling load.
Embodied Carbon
- Specify low embodied carbon materials where possible.
- Specify locally sourced materials where possible.
- Undertake early Life Cycle Assessment, in accordance with the RIBA Plan of Work 2020.
Window Design
- Consider frame to glazing ratio – most of the heat loss is through the frame, so a window with more transoms and mullions will have higher heat losses. Planning context may influence this.
- Consider the materials used for window frames, e.g. timber is more insulating than aluminium or steel.
- Consider the type of glazing.
- Consider the size of opening.
- Consider the ratio of solid wall to glazed area.
- Consider the orientation of window.
- Glazing will perform differently on different elevations. For example:
- South facing windows can reduce heating demand as they enable solar gain during the winter months, and are easy to shade in the summer to prevent overheating.
- North facing windows provide good daylight, without creating glare issues, but suffer higher heat losses during the winter.
- Windows on east and west facades experience greater fluctuations as they experience more extreme conditions at different times in the day, i.e. morning glare on east elevations and afternoon glare as well as overheating from west facing windows.
- Consider position of window within the wall construction:
- The position of a window within the external wall zone can also affect performance, particularly around the perimeter. To avoid thermal bridging around window frames, it is best to locate a window within the insulation zone.
- Consider proportion of solid wall to glass:
- A building should be designed to provide sufficient levels of natural light and ventilation. However, the proportion of glazed elements to solid walls is important when designing a building for maximum energy efficiency.
- Consider direction of opening, e.g. inward opening allows use of external shutters/louvres.
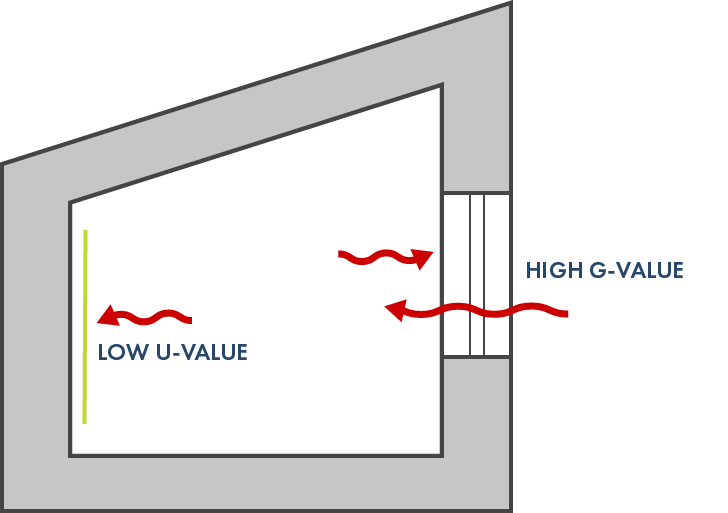
Glazed Element Design
- Frame to glazing ratio
- Frame materials
- Type of glazing
- Size of opening light
- Orientation of glazing
- Position of glazed element within the wall construction
- Proportion of solid wall to glass
- Direction of opening
Treatment of glazed elements
- For natural light
- For passive solar gains
- To avoid overheating and excessive eat loss
- For natural ventilation
- For views
Natural Light
- Building type: direct sunlight for passive solar gains in winter can reduce heating loads for houses; whereas office buildings require light rather than solar gains to avoid glare issues and overheating.
- Poor design of natural light can result in greater energy demand.
- Light shelves or mirrored louvres can boost natural light, particularly in deep plan buildings, or in rooms with single aspect glazing. These can be combined with solar shading devices.
- In deep plan buildings, consider using rooflights, sun tubes, courtyards or atria to provide additional natural light.
- Space planning, including building service zones, should take into consideration the use of solar gains. For example, in dwellings, the living spaces might be located on south-west side of the building, with service zones located to the north and bedrooms facing east.
- Fully glazed facades present a challenge to the environmental design of buildings. They are usually highly energy intensive, due to overheating and glare issues in summer months and thermal losses in winter, meaning the internal environment must be artificially controlled for most of the year.
Cooling
Consider passive design features to reduce the need for mechanical cooling and air-conditioning, such as:
- Water features
- Vegetation on or around the building – e.g. summer solar gain can be reduced by trees. Vegetation has the added advantage of improving air quality and absorbing sound.
- Shading devices (that don’t compromise daylighting).
- Deep window reveals
- Recessed balconies
- Roof overhangs
- Building form
- Courtyards
Energy
- Consider building footprint and spatial requirements – the bigger the building, the greater the energy consumption. Efficient space planning is key.
- Use performance targets for energy consumption, embodied carbon, water use and wellbeing as set out in the RIBA 2030 Climate Challenge.
- Utilise Passive House Planning Package (PHPP) design tool.
- Use Good Homes Alliance (GHA) Overheating Tool (for new homes).
- The design of glazed elements will affect the heating and cooling demand.
- Use CIBSE TM54 methodology to accurately predict energy demand where possible.
Water
Mitigate flood risk through Sustainable Urban Drainage Systems (SUDS), such as:
- Green roofs and living walls
- Soft landscaping areas
- Permeable paving
- Soakaways and swales
- Ponds and wetlands
Also:
- Consider rainwater harvesting
- Consider greywater recycling
Ventilation
Ventilation strategy is affected by:
- Site location, e.g. in urban or polluted areas, natural ventilation may not be appropriate.
- Site layout.
- Building orientation.
- External spaces.
- Relationships to other buildings (particularly in urban environments) e.g. avoid creating wind tunnels.
- Vegetation on and around building.
- Materials used on and around building.
- Building air tightness – for use of MVHR systems, 3 m3/m2h @50pa is recommended
- Use of thermal mass.
- Equipment used within the building.
Heating
The heating demand of a building is affected by the following passive design factors:
- Building type
- Location, including altitude and local climate
- Building shape or form
- Inclusion of features such as balconies, external porches
- The amount and type of glazing
- Levels of insulation in building fabric
- Airtightness of building envelope
- Ventilation design
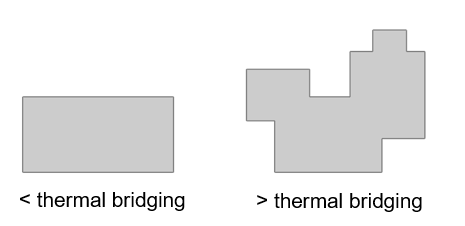
Simple building form = fewer junctions = less opportunity for thermal bridging + better form factor
Acoustics
Incorporate acoustic solutions into the design of a space – acoustic solutions can either blend in to a space or stand out as design features.
Indoor Air Quality
Indoor Air Quality (IAQ) refers to the air quality within buildings and relates to the health and comfort of building occupants. Understanding and controlling common pollutants indoors is critical to ensuring IAQ promotes good health and wellbeing for building occupants. Appropriate levels of ventilation will help ensure good IAQ. Internal finishes, fixtures and fittings will affect IAQ.
- Affects health and comfort of building occupants
- Can be controlled by understanding sources of pollutants
- Appropriate levels of ventilation needed
- Affected by internal finishes, fixtures and fittings
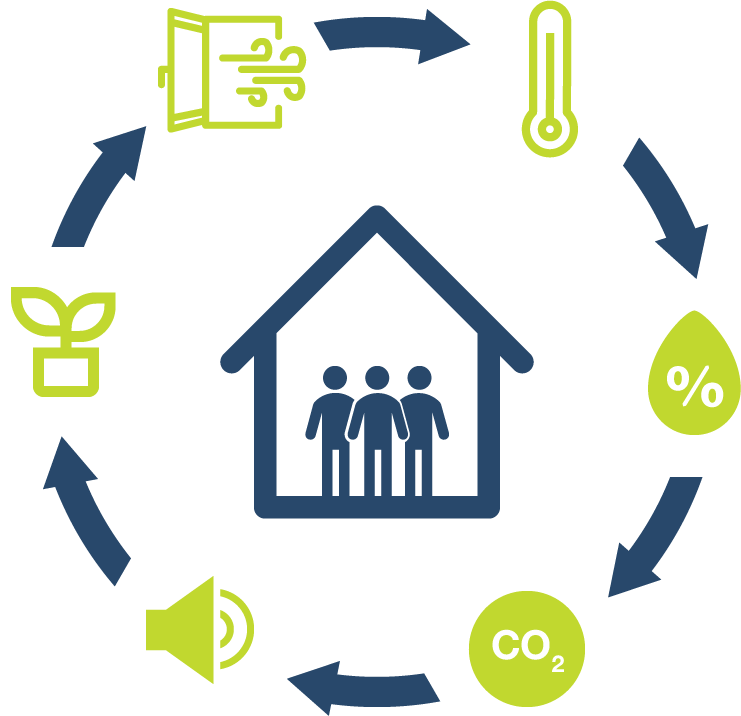
Vertical Movement
Prioritise use of staircases for vertical circulation where possible for health and wellbeing reasons and to reduce energy consumption from use of electrically operated lifts. This can be achieved through:
- Location of staircases (in a more prominent position than lifts, e.g. close to main entrance/reception).
- Use of attractive features on staircases, such as windows or artwork.
- Aesthetics — design that entices people to use the staircase, through the temptation of an interesting journey through a building, for example.
- Width of staircase
- Good lighting
- Use of views
- Use of biophilic elements
- Posters reminding occupants of health benefits
Construction Method
While the choice of construction method is not specified for Active Buildings, the ease of achieving the required insulation thickness, airtightness and insulation continuity should be considered when making the decision on construction types. An engineered solution, manufactured off-site using modern methods of construction (MMC), is likely to offer the best performance, but if this is discounted for financial and/or other reasons, then a high level of site supervision will be essential to ensure the performance levels of the building envelope are achieved (and is highly recommended whatever the construction method). MMC have benefits that align with Construction 2025 targets, including:
- Faster construction time
- Reduced construction costs: reduced labour hours and less construction site overheads
- Improved construction quality – controlled environment
- Improved health and safety: factory environment = safer working conditions, less work at heights, less weather impacts
- Lower impact on the environment – less environmental impact, less waste generated
However, MMC is not without its issues and care must be taken in detailing MMC constructed buildings, particularly the junctions between modules or panels and junctions with other construction techniques, to ensure the factory quality is continued into the site works and final installation.
Comparison of typical construction methods (Source: How to Build a Passivhaus: Rules of Thumb)
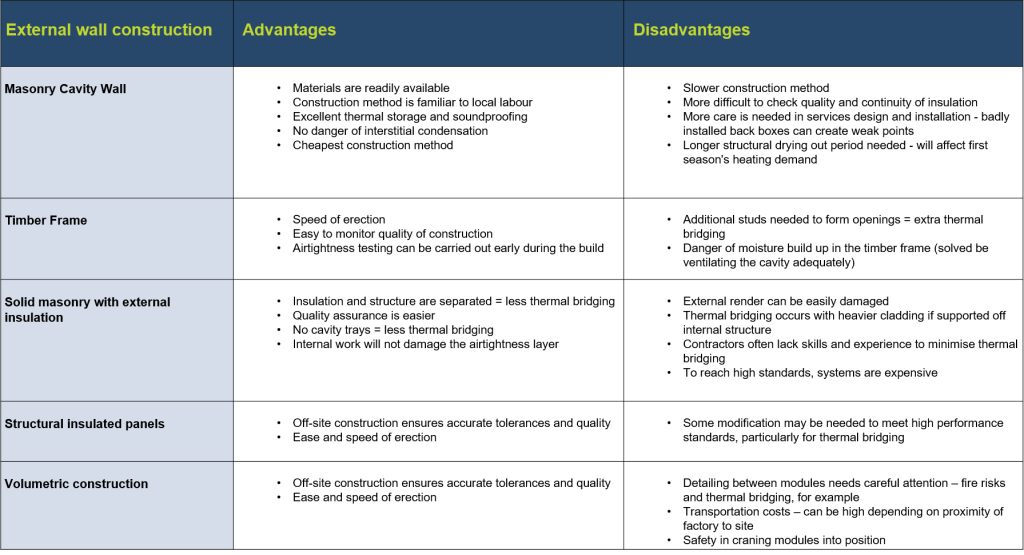
Construction Methods used in Active Building projects to date:
- The POD – Structurally Insulated Panel System (SIPS)
- Active Classroom – Steel-framed panelised system
- Active Office – Steel-framed modular system
- Active Homes Neath – Timber frame and SIPS
Advice
- Close quality assurance (QA) monitoring on site is essential to ensure:
- Details are constructed correctly, particularly to avoid thermal bridging and excessive air leakage
- Any substitution of specified materials or systems align with the Active Building principles
- Undertake in-situ thermography and air-tightness testing both during and post construction to identify any significant thermal bridging issues that could impact on the building’s performance
- Notes of caution:
- Conflicts: views, site orientation, shading, window positions, areas of glazing, acoustics, IAQ
- Consideration of how users operate their buildings, e.g. opening windows
- Available fuel sources – most appropriate solution
- Access to local contractors that can build systems specified: < risk = < cost + < failure
- The need to get specification and detailing right at the start of a project
- The most cost effective way of working, e.g. it is better for the Architect to collaborate with the contractor
- Are materials and construction methods chosen more expensive to build?
- Embodied carbon of materials and construction method
- Are materials selected readily available? And is there a local supply chain in place to deliver the chosen construction method?
- Is construction method suitable for adaptation, e.g. how to maintain airtightness when undertaking future renovations, end of life (design for deconstruction?)
- When specifying solutions such as mechanical ventilation with heat recovery (MVHR), schedules for changing air filters for example need to be included in the building documentation
- Site location and selection of appropriate solutions accordingly (According to the Met Office, there are 11 climate zones in the UK)
- Possibility of future developments impacting on design (e.g. solar energy being blocked by a new building in the future)
Resources
- Clegg, P. Bradley, K. Fielden, R. Gething, B. 2007. The Environmental Handbook. Right Angle Publishing Ltd, London, UK.
- Dollard, T. 2018. Designed to Perform. RIBA Publishing, London, UK.
- Evans, H.M.A. 2016. How Buildings Work. RIBA Publishing, RIBA Enterprises Ltd, Newcastle-upon-Tyne, UK.
- Pelsmakers, S. 2015. The Environmental Design Pocketbook, 2nd Edition. RIBA Publishing, London, UK.
- Roaf, S. 2013. Ecohouse: A Design Guide, Fourth Edition, Routledge, Oxon, UK
- LETI Climate Emergency Design Guide
- RIBA 2030 Climate Challenge
- RIBA Plan of Work 2020
- White Arkitekter
- Building Better Building Beautiful Commission Living with Beauty
- Passhivhaus Planning Package (PHPP)
- Good Homes Alliance – Overheating in New Homes
- CIBSE TM54
Technology Showcase:
Foundations
Steel Screw Piles
Steel screw piles are a versatile, environmentally friendly and cost-effective foundation technology, ideally suited to lightweight structures; and are faster to install than most other foundation solutions. They are typically manufactured from high-strength steel using varying sizes of tubular hollow sections for the pile or anchors shaft.
For an example of screw piles in action, check out our Active Classroom Case Study.
Benefits of Steel Screw Piles:
- Quick to install – saving time, money and carbon
- No concrete or curing time – enables faster commissioning of sites
- Flexible design – frames can be designed to bridge services and other obstructions, allowing areas with congested services, for example, to be built on
- Installation in low temperatures is possible – no down time, unlike concrete
- Cost effective solution in soft ground, where traditional piling is more expensive and concrete is technically unsuitable
- Sustainable – they are removable and reusable
- Minimal vibration – working next to existing buildings is no issue
- No excavations or spoil to cart away – saving money (particularly if there is contaminated ground), transport, carbon
- Minimal noise – dependant on excavation, but most installs below 80db
Low Carbon Concrete Products
Concrete is the most widely used man-made material on earth; and cement, one of the key ingredients of concrete, is the source of about 8% of the world’s carbon dioxide (CO2) emissions. In fact, it has been reported that, if the cement industry were a country, it would be the third largest emitter in the world – behind China and the US.
There are two ways to significantly reduce the embodied carbon of concrete – one is to use a cement substitute and another is to reduce the carbon emitted of the manufacture of concrete.
Here are some examples of available low-carbon concrete products:
- Hanson EcoPlus® Range – sustainable concrete solution that replaces up to 70% of Portland Cement with Hanson Regen GGBS (Ground Granulated Blast Furnace Slag). For more information you can book a free CPD seminar here.
- The process of manufacturing concrete is highly energy intensive and the second largest contributor to carbon in concrete products. An innovative project in Port Talbot, South Wales, is trialling generation of green hydrogen (via a wind turbine) to use as an alternative fuel source in the manufacture of concrete that utilises GGBS.
- Cemfree Concrete – an Alkali-Activated Cementitious Material (AACM) using GGBS and Pulverised Fly Ash (PFA) to create a binder to replace cement.
- Carbicrete – a Canadian company who combine cement substitutes, such as GGBS with carbon capture technology, where the carbon emissions from their industrial process are captured, hence reducing the amount of emissions being pumped into the atmosphere. Their future plans include use of direct air capture (DAC) to draw CO2 from the atmosphere.
- Cenin Renewables – at their site in Stormy Down, South Wales, Cenin utilise anaerobic digestion, wind and solar energy generation to manufacture ultra-low-carbon cement substitutes using recycled materials.
Building fabric
Reducing Thermal Bridging
Schöck Isokorb® has been designed to thermally separate elements, such as balconies, parapets or canopy roofs, acting as part of the thermal insulation, while at the same time forming part of the structure. As such, the Isokorb ® products can significantly minimise thermal bridging issues.
Keystone Hi-therm+ Lintels are thermally broken steel insulated lintels with a Psi value of 0.3 – 0.6 W/m2K, significantly lower than a standard insulated lintel.
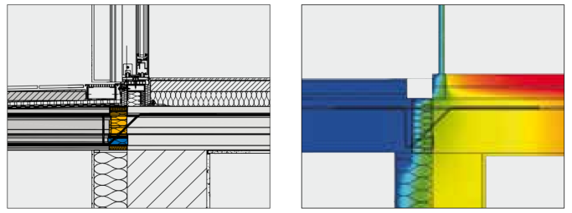
Illustration to show how Schöck Isokorb® minimises thermal bridging (Credit: Schöck)
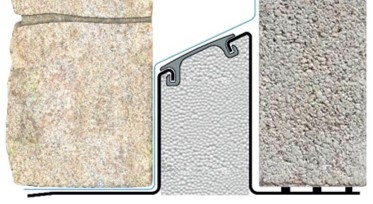
Hi-therm+ lintel by Keystone
Low Carbon Insulation
Many of the mainstream insulation products contain recycled content and/or are recyclable at end of life. ROCKWOOL, for example, use waste material from refurbishment and demolition, as well as off-cuts in their insulation products, which are themselves recyclable. They have a dedicated recycling facility in Bridgend, South Wales, where contractors can recycle unused ROCKWOOL insulation. Here are a few examples of other low carbon insulation products:
Warmcel insulation is manufactured from recycled newspapers, with the addition of naturally occurring mineral salts, which provide fire resistance and fungal/insect protection.
Thermafleece is a natural and sustainable sheep’s wool insulation made in the UK (using wool from UK sheep), comprising a blend of 75% wool with recycled polyester fibres, to provide the enhanced performance of sheep’s wool with durability and sustainability. It can be used in roofs, walls, lofts and floors and can be supplied in different sizes, formats and densities to suit different performance needs.
Natural Insulations – manufacturers of Thermafleece – also manufacture other low carbon insulation products,
including:
- SupaSoft – recycled plastic bottles
- Thermofloc – recycled newspaper
- NatraHemp – hemp fibres and recycled polyester
- Steico – wood fibre from forest thinnings
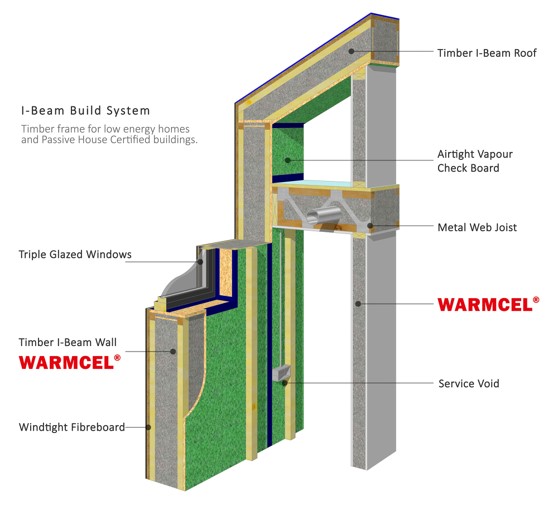
Warmcel insulation detail (Credit: Warmcel)
Inno-Therm®/Métisse® consists of 85% recycled denim/cotton and is recyclable at end of life. It’s manufacture uses 70% less energy than conventional insulation, adding to its low embodied carbon credentials.
Emerging Technology
A London-based company, Biohm, have created a range of bio-based materials, for example mycelium to create building insulation that is naturally fire retardant and removes carbon from the atmosphere as it grows. Mycelium is a biomaterial that forms the root system of fungi, feeds on agricultural waste and in the process sequesters the carbon that was stored in this biomass. It is fast-growing and cheap to produce in custom-made bioreactors.
External Cladding
The lowest carbon cladding systems are those made of natural materials, such as timber. Recyclability of cladding is also an important factor – steel, for example is infinitely recyclable – and the lifetime environmental impacts of any cladding material can be checked via its Environmental Product Declaration (EPD). Cladding materials made out of recycled materials are also increasingly available. One example is recycled plastic cladding by a UK company called Kedel.
Emerging Technology
A German company called Made of Air has developed a carbon-negative bioplastic, using wood waste, that can be
used for external cladding. The material contains biochar, a carbon-rich substance made by burning biomass without oxygen, which prevents the carbon from escaping as CO2.
External Doors and Windows
Glazed elements of a building envelope are often the biggest sources of heat loss, but this can be mitigated through use of high performance glass units and through framing choices. Timber frames, for example, have better insulating properties than metal or uPVC frames. However, timber requires more maintenance than the man-made alternatives. Combining materials such as timber and aluminium can provide a solution to this, and there many manufacturers who provide this hybrid solution. A couple of examples are:
- Norrsken: a UK manufacturer of high performance,double or triple glazed, timber framed windows and doors, with exterior aluminium cladding.
- Vellacine: another UK organisation manufacturing aluminium clad timber framed windows.
This combination is beneficial to overall building performance, as the timber frames have a low thermal conductivity, while the exterior aluminium cladding provides a durable and low-maintenance outer skin. These manufacturers also deploy other measures to further reduce their carbon footprints. For example, to minimise waste, the sawdust from Norrsken’s manufacturing process is used for heating; while Vellacine collect recycled glass from old windows for reprocessing into new glass units.
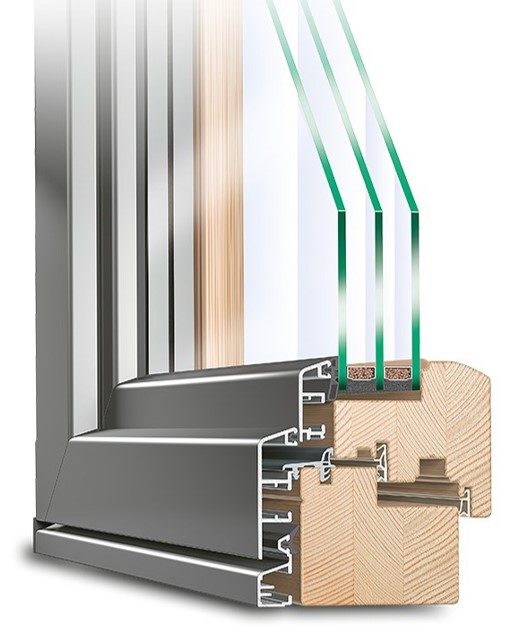
An example of an aluminium clad, timber framed, triple glazed window
Internal finishes for good indoor air quality
Auro Breathable Paints: A wide range of natural plant and mineral based, paints, free from synthetics, pollutants and non-degradable plastics. The use of natural materials creates healthy indoor environments.
Dulux Forest Breath Eco-sense: A high-tech emulsion paint with an air purifying function designed to capture
formaldehyde and a range of other VOCs (Volatile Organic Compounds), as well as effectively killing bacteria. The paint
utilises solvent-free technology which incorporates natural bamboo and charcoal to give it anti-bacteria, anti-virus antibenzene
and anti-formaldehyde properties.