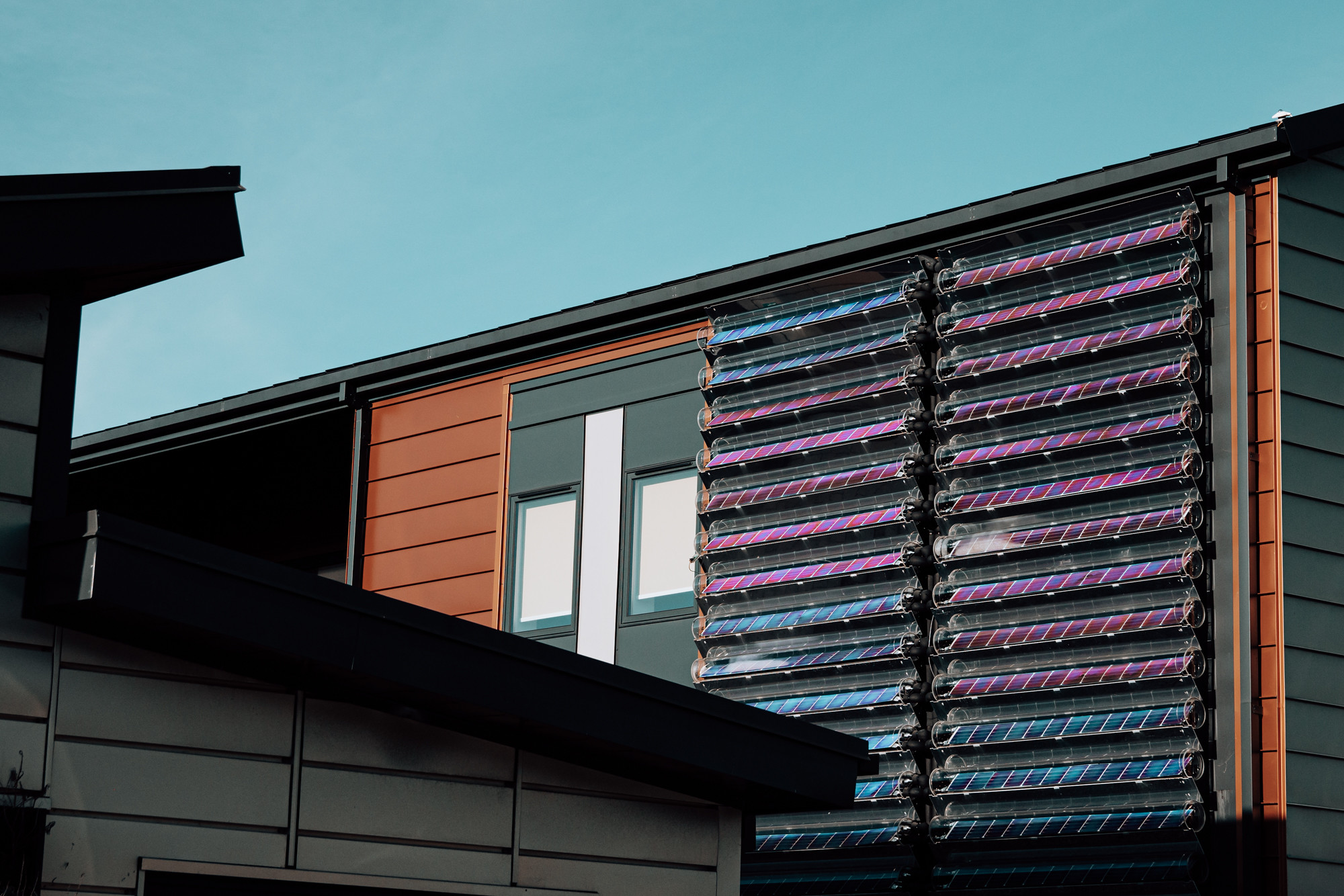
Principle 2: Energy Efficient Systems
Intelligently controlled & energy efficient systems to minimise loads – HVAC, lighting, vertical transportation. Low carbon solutions for heating. Data capture via inbuilt monitoring & standard naming schemas to enable optimisation and refinement of predictive control strategies.
Design Guide:
- Adaptability
- Controls
- Heating
- Ventilation
- Cooling
- Lighting
- Water
- Combining Systems for Efficiency
- Advice
- Checklist for Building Services
- Resources
Adaptability
Design for adaptability, considering medium and long term changes in technology, demographics, climate and future working practices, such as flexible working strategies (e.g. designing for demountable partitioning, highly zoned control systems, modular central and local services).
Controls
Controls are critical to reduce energy consumption, but are often value-engineered out to save costs. It is essential that all parties understand the importance of controls and the role they play in helping to reduce overall operational costs associated with running the building.
Heating
- Model predicted energy loads based on the building fabric and passive design attributes.
- The building’s internal gains must be recognised, from passive solar, occupants and equipment.
- Effects of energy use for cooling, fans, pumps and space heating should be considered.
- Select space heating system to provide the lowest total cost-in-use solution, whilst avoiding gas:
- Prioritise low carbon solutions to be used in conjunction with renewable energy sources where possible. Examples:
- Solar thermal
- Combined heat and power (CHP)
- Air source heat pumps (ASHPs)
- Ground source heat pumps (GSHPs)
- Ground to air heat exchangers (GAHEs)
- Consider low carbon heat networks where practical.
- In selecting a low carbon heating system consider:
- Acoustics
- Availability of skilled installers
- Users knowledge of systems
- Site specific conditions
LETI Future of Heat:
- Fossil fuel free
- Limit peak heat loss to 10W/m2
- Report average carbon content of heat (g/Co2/kWh/y)
- Limit dead leg of hot water pipework to 1 litre
Ventilation
- Natural, mechanical or a combination – mixed-mode:
- Mixed mode is applicable for situations where internal heat gain precludes the use of natural ventilation via passive measures alone:
- Either the whole building could be operated on air-conditioning during peak summer conditions and natural ventilation at all other times;
- Or, air-conditioning could be used in targeted areas that experience consistently excessive heat gain, or are used intermittently (such as meeting rooms), with the rest of the building utilising natural ventilation.
- For mechanical ventilation, specify an MVHR system – to provide the ventilation needs for the building, including fresh filtered air whilst retaining most of the energy that has already been used in heating the building:
- MVHR systems can be used in conjunction with renewable energy generation. To be effective, an air-tightness of <3m3/m2.h @ 50 Pa is recommended.
- Airflows need careful consideration to provide adequate fresh air while preventing draughts. Too much air infiltration could also result in high space heating energy consumption.
- The application of solar assisted ventilation via solar chimneys, wind towers and atria can be considered to promote adequate airflow in deep plan buildings without resorting to fan energy use.
- Consider use of simple ceiling mounted fans to provide air movements in spaces.
Cooling
- Minimise need for cooling through passive design and building fabric.
- Consider appropriate set points for cooling systems – for instance, comfort levels are often maintained or artificially reduced to ‘lower than required’ temperatures in summer, where a set point of 24ºC may be sufficient for occupancy comfort.
- Avoid distributing large volumes of cooled air, to avoid use of significant quantities of fan energy and long lengths of ductwork.
- Low carbon alternatives to conventional mechanical cooling should be considered where the heat gain characteristics are such that a natural ventilation solution will not sustain acceptable comfort conditions throughout the year, such as:
- An indirect evaporative cooling system, where supply water is cooled through a conventional cooling tower. In this system the exhaust air stream is humidified and cooled. This coolth is then transferred to the supply air via a high efficiency heat exchanger.
- Groundwater can be used as a viable cooling source, offering a more stable source temperature than that achieved with evaporative cooling. Water is abstracted from well depths of between 5m and 50m at a temperature of approximately 10⁰C. This application is limited to sites with suitable conditions and approval of local authorities.
- Surface water from rivers, lakes or docks, can offer a possible alternative, depending on site location and approval of local authorities; although, the environmental impact of using such sources for heat rejection must be fully evaluated. While temperature fluctuations in source water will naturally be greater, the cooling effectiveness should be greater than that offered by evaporating cooling techniques.
- Cooling distribution for the above systems is via water circuits feeding chilled ceilings or cooling panels and will need to consider dehumidification due to the presence of a low temperature surface. Chilled ceilings and cooling panels obviate the need for high volume air distribution systems. If only a small part of the building requires cooling, the benefits are marginal if demand can be met with decentralised units.
- Use of night-time purging or north intake bypass systems can reduce the need for mechanical cooling, such as air-conditioning. Overnight purge can reduce accumulative overheating experienced during prolonged warm spells. Care is needed to avoid over cooling during the night, which could trigger heating first thing in the morning. Use of appropriate deadband settings will avoid this issue.
Lighting
- Specify energy efficient lighting systems, for low energy consumption and reduced cooling demand.
- Where possible, consider integration of high efficiency task lighting with scheme lighting to reduce overall demands.
- Incorporate energy saving features into lighting systems to reduce use of artificial lighting, such as:
- Daylight dimming sensors to realise savings from maximising daylighting – lights react to external weather conditions. Where daylight dimming is employed, suitable response times are required to avoid obvious and distracting fluctuations in artificial light adjustments as clouds or other external influences are detected.
- Digital Addressable Lighting Interface (DALI) controls to enable automating control of light fittings dependant on occupancy requirements
- Passive infra-red (PIR) sensors, linking lighting use to occupancy. Where PIR occupancy sensors are employed, appropriate adjustments an options for reducing premature switching off may be required.
- Absence detection which enable users to switch on a light manually when they enter a room, but sensors detect when the room becomes vacant and switches off the lights automatically, linked to pre-set timers.
- Assess visual comfort of lighting installations to ensure the well-being of occupants, taking into consideration aspects such as lighting flicker, glare, aesthetic impressions of internal finishes, etc.
Water
- Prioritise reducing water over recycling it.
- Rainwater harvesting systems have advantages and disadvantages:
- Only consider where viable and economically feasible, for use in landscape irrigation, for toilet flushing and washing machines.
- When using rainwater harvesting, use simple water butts without pumps where possible, to reduce energy demand.
- Rainwater harvesting can reduce the strain on storm water or combined sewers and reduce the amount of mains connected water use.
- Use of rainwater harvesting could increase a building’s carbon emissions compared to mains water supply and will increase maintenance requirements.
- Options for rainwater harvesting:
- Gravity-fed system – water is collected from the roof, taken to a tank in the ground, pumped into a header tank at high level and gravity-fed down to devices. Spare renewable energy could be used to charge the header tank when available, although power consumption of the water pump can be relatively minor in comparison to other building systems.
- Indirect feed system with a break-tank which allows small amounts of water to be drawn off without pumping.
- Greywater harvesting involves collecting water from baths or showers for use in toilet-flushing.
- Waste energy from showers can be harvested using a simple heat exchanger around the incoming mains cold water supply. This can be simple to implement if designed in to the system early enough.
- Specify water saving devices, such as:
- Water-saving shower heads – aerated or non-aerated
- Low-flush or dual flush toilets
- Sensor operated controls for taps and toilet flushing
- Knee or foot operated controls
- To reduce energy required for hot water supplies, always ensure pipes and tanks are well-insulated to reduce losses.
Combining Systems for Efficiency
- All building services should work together in one optimised system, optimised to make the best use of available energy, whether generated on-site or grid-supplied, and be capable of distributing the energy in the most appropriate and efficient way.
- Robust system controls are essential to ensure systems operate effectively.
- Embedding metering devices and sensors into energy systems is essential to:
- Enable issues to be identified and resolved quickly.
- Provide feedback on actual building performance.
- Enable the development of planned maintenance strategies.
- Enable optimisation of system to save energy and operational carbon.
- Enable the development of predictive control strategies.
- Educate building occupants on their energy consumption and how the energy used is provided.
- Enable controlled import and export of energy, based on factors such as grid Carbon Intensity (CI) or cost.
- Careful commissioning is essential to ensure all systems installed operate efficiently and are optimised to provide the environment required, while minimising energy use.
- Building users must be trained in the correct operation of the installed environmental systems and must understand the overall energy strategy and Active Building design philosophy.
- Building user manuals should be provided.
- Part of the handover strategy should include a building review (similar to an MOT for motor vehicles) after the first year of operation.
Advice
- Before embarking on design of building systems, it is essential to know the occupancy profile.
- Ensure low carbon systems selected fit into the overall energy strategy for the building.
- Utilise ‘CIBSE TM54: Evaluating Operational Energy Performance of Buildings at Design Stage’ to accurately predict energy consumption.
- Implement design measures and key performance indicators described in the LETI Climate Emergency Design Guide.
- Never underestimate the size of ductwork and other building services equipment.
- Air flows in buildings are complex and should be modelled as accurately as possible in design stages.
- Data sheets for technologies are not 100% reliable. They often do not consider how particular technologies or equipment work as part of an overall system. Bear this in mind when specifying.
- Position, size and layout of plant rooms is critical to the successful operation of the building. Plant rooms should be fully modelled during design stages, including air flows around equipment, maintenance access, etc.
- Consider exposed services – better maintenance access, more efficient design and installation, less materials (no ceilings).
- When modelling energy consumption, include all consumers, including network sockets, fire alarm panels, comms panels, etc – these are often not factored into energy modelling but can be high consumers when accumulated.
- When modelling energy consumption and generation, the load profiles (when energy is used, not just total requirements) is as important as the total energy consumption. Time shifting possibilities for significant loads, bearing in mind any roundtrip efficiencies or sacrificial losses (thermal decay from hot water tanks for example), should be considered.
- Incorporate plenty of metering. This is essential for data capture for fault detection, maintenance strategies, etc. As a minimum, regulated and unregulated loads should be understood, so that heating and hot water demand can be compared to estimated, for example. Loads such as EV charging should also be separated, again to ensure that the building operation can be compared to design performance. Ensure metering is installed and commissioned correctly.
- It is highly advisable to appoint a Quality Assurance (QA) professional to monitor site activities, oversee installation and ensure building services are installed correctly in accordance with design specifications.
- Employ HVAC Control Specialist as early in the project as possible.
- The HVAC Controls Contractor should provide all aspects of the control systems, hardware and software for the MEP controls.
- At commissioning stage, the HVAC Controls Contractor must test connectivity between systems and ensure that data is being captured as specified.
- Communication is critical in Active Buildings – ensure enough network sockets are included.
Checklist for Building Services
To avoid spatial and routing issues that could affect the overall architectural design strategy and aesthetics, building services must be considered early in the design process (RIBA Stage 2 – 3) in an Active Building, as services are integral to the overall holistic building design. If not considered properly, this could result in aesthetic compromises and sub-optimum building performance. Mechanical and electrical schematic layouts will not provide sufficient detail to enable scrutinization of system design. Considerations for designers include:
- Ease of access for maintenance, upgrading, adaptability and renewals, applicable to:
- Cable and ductwork runs
- Connection and metering equipment
- Energy storage systems
- Control boxes
- Design of cable routes and how these can be integrated into the overall architectural design:
- Small power
- Communications/data
- Fire
- Lighting
- Length and optimal routes for cables and ductwork
- If using heat pumps, consider:
- Location:
- ASHPs external, GSHPs can be located internally
- Ease of access for maintenance
- Distance surrounding heat pump
- Acoustic considerations
- Away from vegetation
- Away from direct sunlight
- Design of base
- Length of pipe runs
- Insulate pipe runs with weatherproof pipe lagging
- Location:
- Is surge protection needed? If so, where will the surge protection device (SPD) be located?
- Is lightning protection needed? If so, where will it be located?
Resources
- Clegg, P. Bradley, K. Fielden, R. Gething, B. 2007. The Environmental Handbook. Right Angle Publishing Ltd, London, UK.
- Evans, H.M.A. 2016. How Buildings Work. RIBA Publishing, RIBA Enterprises Ltd, Newcastle-upon-Tyne, UK.
- Pelsmakers, S. 2015. The Environmental Design Pocketbook, 2nd Edition. RIBA Publishing, London, UK.
- LETI Climate Emergency Design Guide.
- RIBA 2030 Climate Challenge
- NHBC The Future for Home Heating – life without fossil fuels
Technology Showcase:
- Heat Pumps
- Mechanical Ventilation and Heat Recovery
- Electric Infrared Heating
- Convection Radiators
- Low Carbon Heat Networks
- Hydrogen
- Energy Efficient Hot Water
Heat Pumps
Heat pumps use the refrigeration cycle to extract heat from either the ground, air, or water at a low temperature, absorbing the heat into a fluid. This heat can then be used to heat radiators, underfloor heating systems, or warm air convectors, and hot water. The system selected will depend on site specific conditions and financial or legislative constraints. Although they use electricity, the power input required is typically between half to a fifth of the available heat output. This is known as the coefficient of performance (COP) and is used as a measure of efficiency of a heat pump. The COP will vary depending on temperature input and output and the seasonal performance factor (SPF), which is the average COP of a heat pump over a full heating season. Ground source heat pumps tend to have a higher COP than air source heat pumps, for example, as the temperature of the ground is more stable and generally warmer than ambient air temperature.
COP = useful heat output (kW) ÷ electrical power input (kW)
The low electrical consumption of heat pumps in relation to their thermal output is further improved when used in conjunction with renewable electricity generation, making them a low energy and low carbon choice for heating buildings.
Air Source Heat Pumps (ASHPs)
ASHPs use the refrigeration cycle to absorb heat from outside air at low temperature into a fluid. This fluid then passes through a compressor where its temperature is increased, and transfers its higher temperature heat to the heating and hot water circuits of the building.
Key Design Considerations:
Space, location, acoustics, heating system
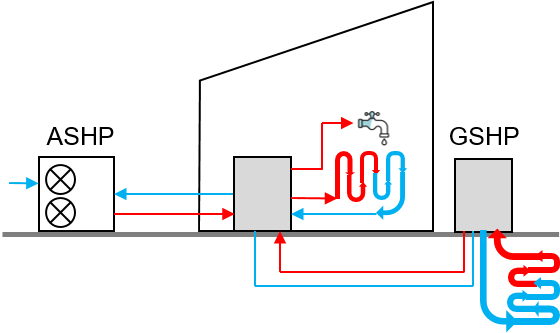
Diagram of ASHP or GSHP connected to heating and DHW circuit
Ground Source Heat Pumps (GSHPs)
GSHPs use the refrigeration cycle to harness heat from the ground by pumping water through pipes within the ground to provide space heating and hot water.
Key Design Considerations:
Ground conditions, available area, heating system
Ground to Air Heat Exchangers (GAHEs)
Also known as earth tubes, GAHEs are used to pre-temper ventilation air by drawing air through underground pipes at a depth of 1.5m – pre-cool air in summer and pre-heat air in winter, using the near constant temperature of the ground.
Key Design Considerations:
Location of air intake pipe, ground conditions, required flow rate, pipe length, pipe diameter, condensate trap location
Mechanical Ventilation and Heat Recovery (MVHR)
An MVHR system provides fresh filtered air into a building whilst retaining most of the energy that has already been used in heating the building, as well as providing the ventilation needs of an Active Building. An MVHR system can provide a constant supply of fresh filtered air, maintaining the air quality whilst being practically imperceptible. It works by simply extracting the air from polluted sources such as kitchens, bathrooms and toilets, and supplying air to occupied spaces. Extracted air is taken through a central heat exchanger and the heat recovered into the supply air. This works both ways, if the air temperature inside the building is colder than the outside air temperature then the coolth is maintained in the building.
Decentralised MVHR (dMVHR)
Decentralised MVHR systems are typically installed through outside walls, resupplying fresh, tempered air into the room they are extracting from. They are generally small, compact units, using less energy than a standard MVHR system and have minimal to no ductwork, reducing restrictions on their locations and making them ideal for retrofit situations. Their flexibility in location also reduces time, disruption and costs associated with installation.
Examples include:
- LUNOS
- See p.42 – 49 of Passive House Plus magazine, issue 34, for an example of use in a deep retrofit scheme
- Ecostream
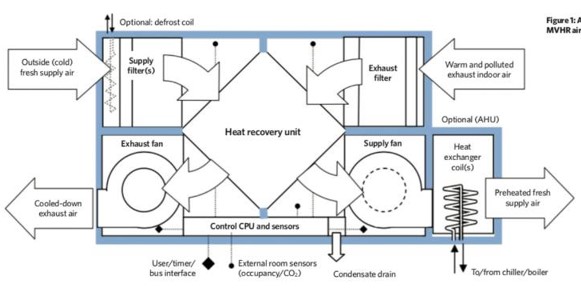
Combined MVHR with ASHP
The Genvex Combi 185 unit combines MVHR with an ASHP to provide space heating and to supply a 185L integral water tank, which also has a 1kW immersion heater for fast delivery of hot water. This unit is Passive House Institute (PHI) certified for a heat recovery efficiency of around 70%.
The integrated ASHP prioritises the production of domestic hot water, providing space heating once the hot water cylinder has been fully charged. The hot water cylinder can be connected to solar thermal generation.
Another example of a combined unit is the Pichler PKOM4.
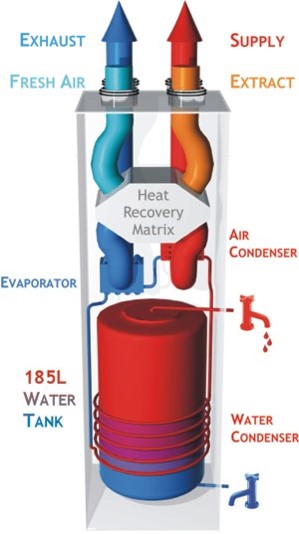
Electric Infrared (Radiant) Heating
Radiation emitted from the heating unit travels through the air until it hits an object or person, at which point the object or person absorbs the radiation, causing molecules to vibrate, producing heat. As radiant panels heat objects or people in this way, rather than heating the air, they can be more efficient in large volume spaces.
There are no direct carbon emissions from electrical radiant panels and their electrical consumption can be supplemented with solar PV.
Panels are typically located on walls or ceilings.
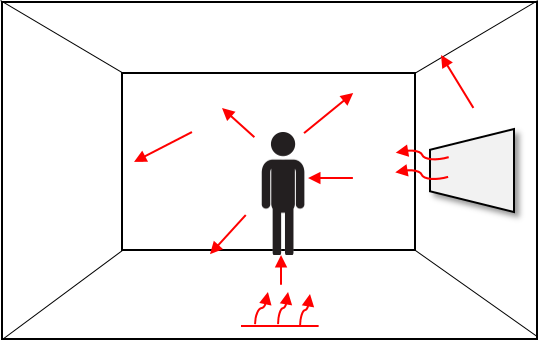
heating the objects they come into contact with, including walls and people
Emerging Technology
Researchers at SPECIFIC have developed a novel form of electric resistive heating that can be incorporated into floor panels, providing a low-temperature electric underfloor heating system. It can also be used in wall panels. The technology uses a conductive coating applied to floor or wall panels, which simply heats up when an electric current is passed through the coating. The coating is applied to a substrate using a screen printing process at SPECIFIC’s Pilot Manufacturing Resource Centre.
Significant work has been undertaken at SPECIFIC to develop suitable control systems to enable the heating panels to be incorporated into the overall energy strategy for a building. The key benefit of this technology is that the modular nature of the printed panels enables them to be used in raised access floor systems, while maintaining the flexibility of the raised access floor.
Note: This technology is not yet commercially available.
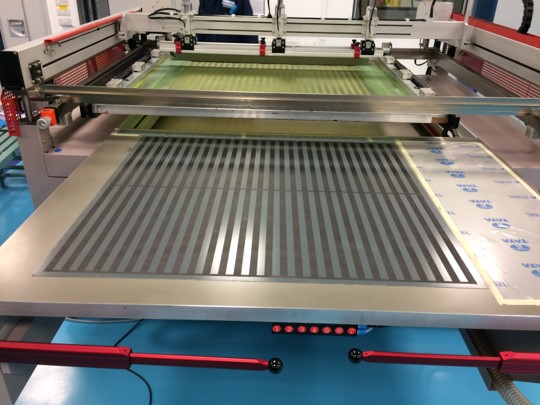
A note about underfloor heating
Traditional warm water underfloor heating systems, when used in conjunction with an air source heat pump (ASHP) and/or rother renewable energy sources, such as solar thermal, can reduce energy consumption and running costs of heating systems. Heat pumps are particularly efficient when operating at the low temperatures and low flow rates required for underfloor heating systems.
Companies, such as Nu-Heat offer a range of different underfloor heating systems suited to different types of construction and different user requirements, including electric systems.
Low temperature, fan assisted convection radiators
Fan convector heaters use lower temperature and less water than conventional radiators, meaning they are an ideal system to use in conjunction with heat pumps.
An example of a low temperature, fan assisted radiator is the Dimplex Smartrad. To provide space heating these utilise a compact, high efficiency heat exchanger and intelligently controlled fan to assist convection and heat delivery. In conventional radiator systems, the water temperature is reduced for thermal efficiency, so radiators tend to be oversized to maintain the thermal output required from a lower temperature differential. Fan assisted convectors reduce the need for oversizing, as the fan element increases the thermal output at lower temperatures. Therefore, these heaters can be much smaller than conventional convector radiators with the same level of output.
Low Carbon Heat Networks
The key elements of a low carbon heat network are:
- Ground temperature heat distribution circuit (can avoid expensive insulation to pipework)
- Heating and cooling provided by a heat pump in each building
- Demand side response (DSR) to reduce the cost of electricity and reduce carbon emissions at peak times
- Thermal energy storage to extend the benefits of DSR
- Opportunities for integration of waste heat from buildings or processes that reject heat above ambient ground temperature
- Flexibility to expand or contract the network to meet changes in demand
Hydrogen
Hydrogen is deemed a potential low carbon heat source as it emits no CO2 at the point of combustion. However, there are still (2020) significant challenges with use of hydrogen, some of which are listed below:
- Burning hydrogen in the air may produce up to six times the level of NOx emissions than burning gas
- Upgrading the exiting natural gas network would incur huge cost and disruption. The existing high pressure pipelines are not suitable for hydrogen, so will need to be upgraded or replaced
- Safety concerns – potential of hydrogen leaking from pipe network
- Hydrogen meters would need to be installed in buildings
- Hydrogen is only a low carbon heating source if the hydrogen is generated from renewable energy sources
- Hydrogen boilers do not yet exist (2021), although Bosch have launched a prototype hydrogen gas boiler
Further information:
Energy Efficient Hot Water
Hot water systems designed to optimise energy consumption and increase the utilisation of renewable energy through intelligent control strategies are now available. One example of this is the Mixergy tank, which can utilise different energy sources and make use of time of use tariffs to maximise benefits to consumers and to the energy grid infrastructure.
Energy efficiency is achieved by only heating the water needed, rather than heating the whole tank. This in urn allows faster delivery of hot water. The tank can be heated from a variety of energy sources – conventional gas or electric boilers, solar PV, solar thermal or heat pumps. They can be controlled either via the Mixergy App, or are compatible with other smart home management devices.
Further information can be found here: Mixergy.
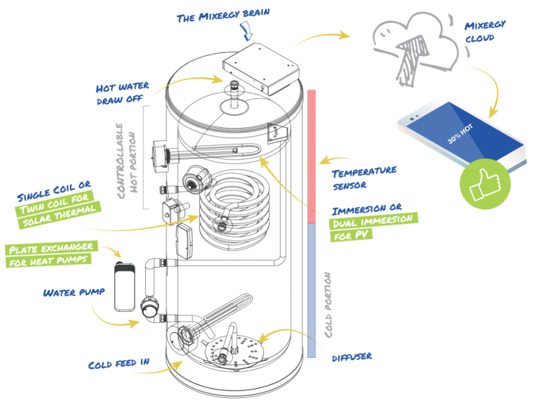