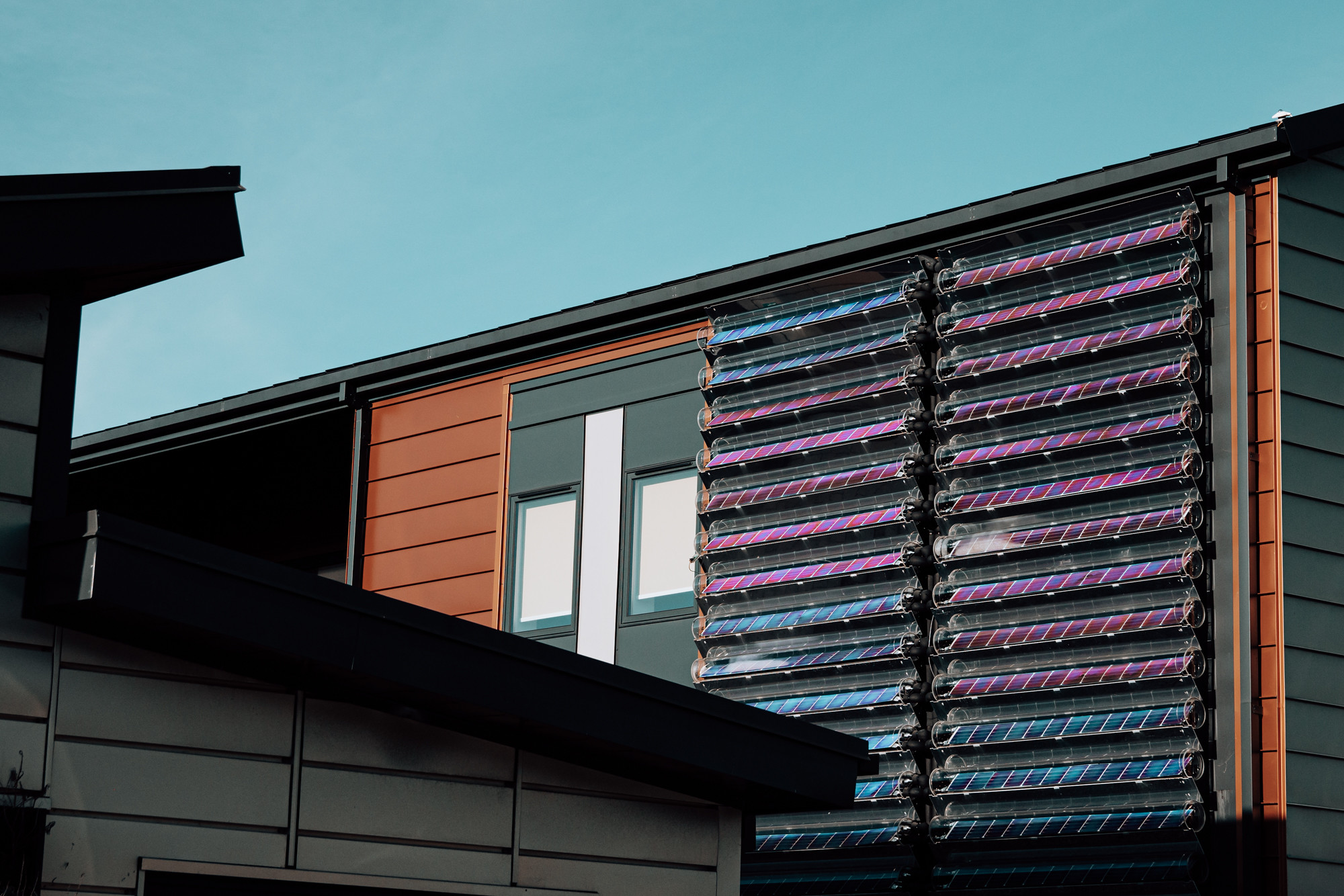
Principle 4: Energy Storage
Thermal and electrical storage to mitigate peak demand, reduce the need to oversize systems, and enable greater control, with a view to supporting the local infrastructure through time shifting of demand and controlled export. Storage will also enable flexible control for virtual power plant integration.
Design Guide:
Electrical Energy Storage
- Electrical storage is essential to enable optimum use of electricity provided by renewable energy generating technologies and the grid.
- The most widely used form of electrical storage is batteries (refer to Active Building Technology Showcase).
- Electrical storage enables:
- Management of the lulls (periods with little or no solar energy production) and slews (short-term changes in either supply (a lot of solar energy) or demand (a large power draw))
- The maximum use of renewable energy, storing the energy as it is generated for when it is needed – hence reducing the amount of grid-electricity needed and hence reducing energy bills
- Building owners to choose when to use their solar energy and when to export it to the grid, demand shifting grid-connected electricity usage, through a smart control system
- Reduced pressure on the grid
- Export of both spare power being generated at a point in time, or previously stored power to time-shift export, enabling peak shaving or tariff benefits, in the right circumstances and with agreements could mitigate the high cost of local grid upgrades required for high power, but intermittent load profiles. Energy storage acts as a buffer to enable this – careful consideration of this operation is required.
- Electrical storage can store energy for relatively short periods of time (up to 2 days) but relies on a smart system combining storage with demand-side response, shifting load profiles, for longer periods.
- The energy storage control system will determine when to switch appliances on, when to charge electric vehicles, the optimum time to charge the batteries and when to export energy to the grid.
- When designing energy storage, future scenarios could consider the use of EVs as an additional route to provide flexible storage (see Principle 5). Smart charging and bidirectional charging functions enable ‘vehicle-to-grid’ (V2G) technology to allow EVs to export electricity back to the grid. V2G is unlikely to be widely available until after 2025.
- Location:
- Close to generation to minimise losses
- Safe, self-contained area
- Ventilated space to avoid heat build-up
- Spatial requirements for storage and associated systems, including space needed around equipment.
- Electrical storage technologies have high capital costs, so systems should be sized carefully, according to the purpose of the storage, e.g.:
- For self sufficiency
- To optimise electricity use
- To reduce operational carbon
- To take advantage of time-of-use tariffs or trading opportunities
- The efficacy of storage solutions can be measured according to different criteria:
- Energy density (or how much energy is stored per kilogram of storage system), although for static applications (buildings), the volume of storage is likely to be more important than weight
- Storage form factor
- Modularity
- Instantaneous power draw (also tied to inverter power)
- Full roundtrip efficiency (amount of energy available for use compared to energy put in, including inverter losses, as well as losses related to battery chemistry)
- Lifetime (how many cycles of energy storage can be delivered before the system needs refurbishing)
- Maximum rate at which energy can be pumped into or out of the storage system (power per kg)
- Duration for which energy is stored in the system; cost of the system; and safety
Specification decisions will include:
- Cycle lifetime
- Maintenance requirements
- Roundtrip efficiency
- Self-discharge rate
- Weight
- Cost
- Energy density
- Instantaneous power available
- Controllability
- Capacity required
Summary of electrical storage benefits
- To reduce the capacity of renewable energy generation needed to help achieve carbon reduction targets by improving the utilisation of the intermittent renewable energy generation, i.e. a smaller PV array could be considered, if used in conjunction with storage. However, from an economic viewpoint, it can be better to oversize PV array (and limit peak output via inverters), rather than install a small PV array with battery storage (in 2020).
- To reduce operational carbon by importing electricity when the carbon intensity (CI) of the grid is low (regardless of demand) and exporting electricity to the grid when the CI is high. (Note: this could have wider implications on the grid networks if all buildings operated in this way)
- To save money, by importing electricity from the grid when the price is low and using stored electricity or exporting to the grid when the price is high.
- To enable system balancing, storing electricity generated from renewable sources when yield is high and utilising stored energy when generation is low.
- To store electricity during times of network stress or to overcome network constraints.
- To reduce peak loads.
- To improve the utilisation of grid supplied electricity and defer investments in transmission and distribution network reinforcement (wider benefit).
- To provide grid support via frequency response or external control via an aggregated virtual power plant (VPP), in conjunction with an appropriate control system.
Thermal Storage
- Thermal storage should be considered to realise the true benefits of a solar heating system, storing heat generated until the heat is ready to be used.
- There are three types of thermal storage:
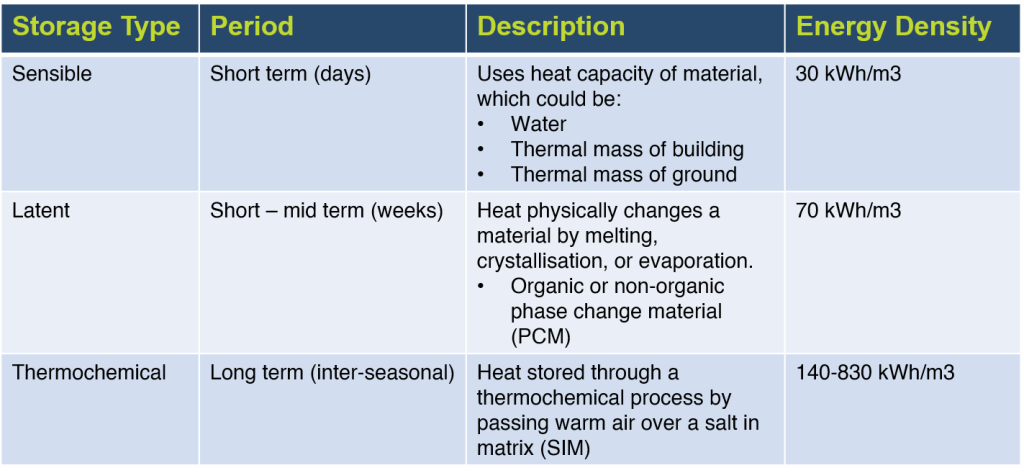
Dos and Don’ts of Solar for Electricity Generation:
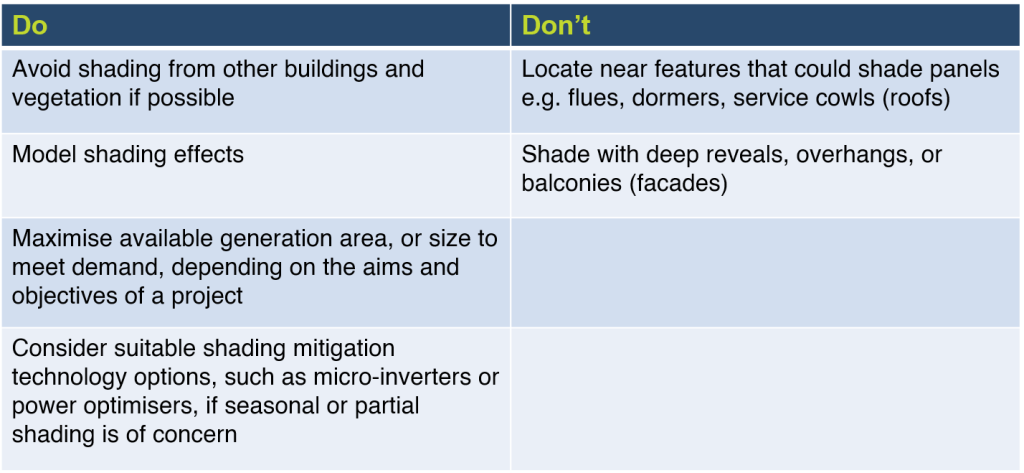
Solar PV choice will depend on:
- Planning constraints
- Aesthetics
- PV area to volume ratio
- Main driver
- Available surface area
- Predicted energy consumption
- Site conditions
- Building type
Dos and Don’ts of Solar for Heat Generation:
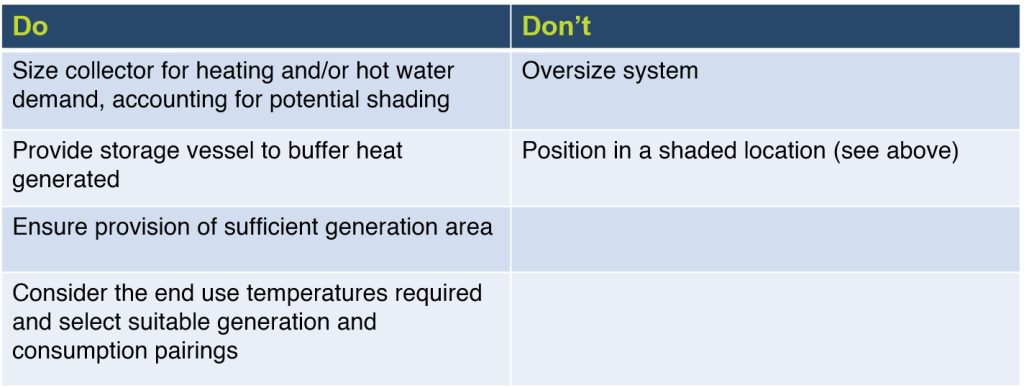
Solar thermal choice will depend on:
- Planning constraints
- Site conditions
- Maintenance requirements
- Predicted energy consumption
- Heating system
- Aesthetics
- Source temperature required
- Building type
- Peak temperature required
- Available surface area
General Considerations for all Energy Storage
- Purpose:
- To assist grid interactions, via time shifting of significant demand or remote control via some grid health measure (frequency, voltage, etc)
- For self-sufficiency/resilience
- Space requirements
- Location:
- Close to generation to minimise losses
- Safe – self-contained area for safety, ventilated space to avoid heat build-up
- For storage such as water tanks, ensure high levels of insulation to minimise heat losses.
Technology Showcase:
Electrical Energy Storage
Electrical storage enables solar energy produced during the day to be stored for use in the evenings and overnight, or to provide higher power than the current PV output would be generating – essentially buffering PV generation. The most commonly used form of electrical storage in buildings is electrochemical, i.e. batteries, the most widely used battery technology being lithium-ion (li-ion). Lithium-ion batteries have developed such that they are virtually maintenance free. The cost of battery storage is rapidly reducing, making it a financially viable solution for buildings. Other emerging battery technologies include:
- Aqueous Hybrid Ion (AHI™)
- End-of-life EV batteries (li-ion)
- Flow batteries
Aquion Aqueous Hybrid Ion (AHI™) “Aspen” batteries were installed in the Active Classroom as part of the construction phase in 2016. These batteries are sealed electrochemical energy storage systems based on a saltwater electrolyte. They are manufactured using earth abundant, non-toxic materials and low cost manufacturing techniques. They were purported to be maintenance-free and optimised for daily deep cycling. This 60kWh installation comprised two 30kWh batteries, consisting of individual modules.
This technology is relatively immature, so should be considered for use in building projects with caution. The particular batteries installed in the Active Classroom were found to be underperforming after two years of operation, so were removed for testing.
In 2020, the Aquion batteries were replaced with twelve 10kWh Redox flow batteries, providing a total of 120kWh. These modular zinc-bromine batteries represent another emerging technology and this was the UK’s first installation of the technology. This installation will test claims that the battery system is capable of delivering 100% of the rated energy every day, without degradation in capacity over a 10-year lifetime.
Further information about the installation can be found here.
Other less widely used forms of electrical storage include:
- Hydrogen fuel cells (if hydrogen is produced from renewables)
- Compressed air
- Flywheels
- Ice
- Electrochemical
- Immersion heaters in combined thermal and electrical systems
Solar Power Diverters for Hot Water
It is possible to divert excess solar power generation to a hot water tank, using a controlling device, such as IMMERSUN or others reviewed here. This enables maximum utilisation of solar power or other renewable energy sources, such as wind or hydro power.
How they work:
PV export is monitored at the meter connection point and the required energy is diverted to the resistive immersion heater to maintain a zero export or zero import scenario. Such diverters work to prioritise direct electrical usage, only storing excess electricity when available and not required as power. Many systems have two controllable outputs – the first to an immersion heater and the second to either a second immersion or another resistive load, such as electric towel rails, swimming pool heaters or electric underfloor heating systems.
Solar Power Diverters can also be controlled to divert excess energy to electric vehicles (EVs). In this instance, they will charge the EV at a different speed depending on the amount of solar energy available. To operate effectively, these need an export in excess of 1.4kW (the minimum EV charger power in the charge protocol). Chargers can be overridden to charge from the grid if charging at a faster rate is required.
Emerging Technology: Cornish Lithim
As mentioned above, the main type of rechargeable battery storage currently used in both buildings and electric vehicles is lithium-ion (Li-on).
A UK company called Cornish Lithium is currently investigating extracting lithium from geothermal brine and hard rock in Cornwall, using innovative mineral exploration techniques, which they claim to have a net zero carbon footprint. Demand for lithium is set to increase rapidly over the next decade to supply electric vehicles and to store renewable power for buildings. This will provide a secure and resilient supply of lithium for the UK, contributing to a sustainable UK supply chain for Li-ion batteries.
The company is currently (2020) constructing a pilot lithium extraction plant, with a view to starting extraction within the next 5 – 10 years.
Sensible Thermal Storage
Heat causes a change in temperature in a body or thermodynamic system. Energy density typically 30 kWh/m3
Often overlooked in favour of Electrical Energy Storage, Thermal Energy Storage (TES) is an important technology for both energy conservation and the efficient large-scale deployment of renewable energy. It successfully addresses the intermittent nature of renewable energy generation by storing excess heat during periods of low demand for use later when demand is high.
Thermal Energy Storage (TES) systems for sensible heat were, until recently (2021) typically diurnal, with the most common examples being water tanks with integral copper coils or heat exchangers. These enable heat generated and stored during the day to be released overnight or the following morning, although some allow longer “periodic” storage. The scale varies from domestic systems storing up to 100 litres for use as domestic hot water (DHW), to district network scale installations containing 10,000 m3+.
The heating system for the Active Office utilises a 2,000 litre water cylinder, which stores heat generated by PV-T tubes and an air source heat pump (ASHP); and enables time-shifting of heating demand, with the capability to charge the tank on sunny days or overnight for use when it is needed to heat the building. A significantly smaller cylinder could be used in an Active House, similar to those used in conjunction with traditional gas-fired boiler systems.
Short term: diurnal
Long term: inter-seasonal
Underground Thermal Energy Storage (UTES)
Underground Thermal Energy Storage (UTES) is where thermal energy is stored in the ground for later extraction using Ground Heat Exchangers (GHE) e.g., ground loops or similar structures. The ground is suitable for thermal energy storage because it has high thermal inertia and, if undisturbed, below a depth of 10-15 m, the ground temperature is only mildly affected by local climate variations and maintains a stable temperature approximating to the local annual mean air temperature.
UTES can retain thermal energy for extended periods of time – inter-seasonal storage is most common, but storage from one year to the next is possible. The thermal energy may be captured from a variety of renewable sources, including ambient air (with or without a heat pump to modify storage temperatures), solar and by-product or waste heat from industrial and other cooling processes, such as from Data Centres.
Using stored thermal energy from renewable sources instead of “newly generated” fossil fuel derived energy is the key to achieving Net Zero Energy Buildings (nZEB) and/or Positive Energy Buildings (PEB), and should be considered an integral part of the UK’s decarbonisation strategy.
Among UTES technologies, Aquifer Thermal Energy Storage (ATES) & Borehole Thermal Energy Storage (BTES) are the most familiar, although Foundation Energy Storage (FES) is increasingly employed, combining energy storage with structure, hence reducing total capital costs. Disused mine workings, as well as pit and earthbank storage, for example ThermalBanks™ by ICAX, are all viable options.
While ATES can be highly effective, it requires suitable hydrology and due to the capital costs can often be hard to justify economically. BTES may be used at a local scale to supply cooling and/or heating to houses and other domestic buildings, at settlement or community scale for districts or groups of houses and at grid scale for larger district heating and cooling networks or commercial and industrial buildings. BTES’s ability to store heat inter-seasonally at scale was first explored in detail in Sweden at the University of Lulea from 1982-1988 where waste heat captured from a steel works was recovered and recycled to heat student accommodation. The following section describes BTES in more detail.
Borehole Thermal Energy Storage (BTES)
Borehole Thermal Energy Stores may be used to provide inter-seasonal heat storage, flexibility, additional capacity and/or load balancing to District Heat and Cooling (DHC) networks. They are ideal to store what would otherwise be waste heat especially from commercial and industrial processes, such as from Data Centres, Foundries, Combined Heat and Power (CHP) systems, or even bakeries and cold stores.
They consist of a series of boreholes identical to those used solely to collect or reject heat, but with the positioning and spacing of the boreholes optimised during the design process, specifically for heat storage.
Case Study: The GeoTermos BTES Headworks
At GeoTermos, in the municipality of Drammen, Norway, there is a project to store solar energy as heat. The system stores energy provided by 150 m2 of solar thermal collectors and 1,000 m2 of PV panels in 100 x 50m boreholes in granitic gneiss rock.
“GeoTermos is expected to return around 350,000 kWh/year in the form of heat at various temperature levels during the heating season.”
Computer aided simulation techniques are used to model the heat flows into and within the store. This both optimises operation and minimises size and installation work to yield considerable savings.
Additional resources:
UTES is not a new concept, but is underpinned by considerable detailed research and experimentation. For example, Professor Hellström of Enviga Geothermal has been researching thermal energy storage since the 1990s and his PhD thesis on Ground Heat Storage can be accessed here. His subsequent work on BTES builds on this work.
Dr Fleur Loveridge of the University of Leeds is a leading UK expert on Foundation Energy Storage. Her belief is that use of the ground as a thermal store in conjunction with a ground source heat pump systems is an essential technology in our route to decarbonising heat and achieving the UK’s Net Zero targets.
Foundation Energy Storage (FES)
Foundations, primarily piled foundations, are being designed to work as Underground Thermal Energy Stores. This is attractive because a building must have foundations so the cost of modifying these to also function as Underground Thermal Energy Stores is a marginal additional cost compared to boreholes which have only one purpose.
The viability of using foundations to provide large-scale inter-seasonal heat storage was explored in detail in the UK between 2012 and 2015. Much of this work was undertaken at Southampton and Cambridge Universities and led to the UK Ground Source Heat Pump Association (GSHPA) Thermal Pile Standard. This work is now in use worldwide.
Screw piles are increasingly being specified for domestic projects and developments to simplify and speed installation especially on brownfield and problem sites and for offsite manufactured homes. They may be modified to become Ground Heat Exchangers (GHE) and then used to create an UTES below the building.
Thermo Screw Pile™ System
[information provided by Enviga Geothermal]
Thermo Screw Piles™ (TSPs) combine the structural functions of a screw pile with the thermal functions of a ground heat exchanger (GHE), in one quick to install, low-cost construction component.
They may be used to create thermally active foundations which both support the building and collect heat from, or reject heat into, the earth below. This arrangement works very effectively to retain heat for later use diurnally; periodically; or, more unusually, inter-seasonally, where surplus heat is stored in summer for use in winter when there is a shortage. Cold may also be stored in winter to provide summer cooling.
Diurnal and periodic thermal energy storage improves energy efficiency generally, especially during the spring and autumn when cool nights are balanced by warm days. However, the ability to store heat inter-seasonally is the key to achieving major energy savings and ultimately to deliver Positive Energy Buildings (PEB).
The system is ideal for storing heat generated onsite, although any source of heat, ideally renewable or recovered heat, can be employed.
Individual buildings may be networked to manage occupancy variation, load diversity, etc.
Key Benefits of Thermo Screw Piles™ (TSPs):
Standard screw piles are ideally suited to lightweight, off-site construction methods and low carbon building design. They are quick and easy to install and remove, saving both time and money. The installation and removal of screw piles causes minimal ground disturbance and piles can often be used again. Their use minimises the amount of excavated material (arisings) to be removed from a construction site and avoids the use of concrete for the substructure, beneficial for both environmental and time reasons.
The addition of an inter-seasonal thermal storage capability to screw piles provides further economic and environmental benefits for little additional cost and effort. The ability to store heat longer than a few days enables Zero Energy Buildings (ZEB) and Positive Energy Building (PEB) to be constructed economically, which has previously been challenging.
Summary of key benefits:
- Cost savings achievable by using the foundations to store renewable thermal energy for space heating and/or cooling contributes to the thermal comfort and affordability of low to zero carbon buildings.
- TSP foundations may be networked to improve diversity and flexibility. They are particularly well suited to modern (5G) networking techniques.
- TSPs have low embodied energy and may easily be reused or recycled.
- Rapid installation, with minimal spoil excavation reduces the construction costs and environmental impact.
- Constant monitoring of the system by intelligent and intuitive controls maintains the equilibrium of the thermal store, meaning it can compensate for variations in thermal load as well as changing climatic and ground conditions.
- Using underground thermal energy storage adds “virtual” thermal mass to low thermal mass construction methods to further improve operating efficiency.
- TSPs replace much if not all the concrete used in the construction of the buildings substructures.
Keltbray HIPER® Piles
A collaboration between Keltbray Piling, Converge, DB Group and Arup have developed a hollow pile system to combine load bearing qualities of traditional concrete piles, with less and lower carbon materials, and energy generation.
The HIPER® (Hollow, Impression-enhanced, Precast, Energy-generating and Re-useable) Pile uses a hollow pile design and light-weight cement-free concrete to provide the same shaft-bearing capacity with fewer or narrower piles. The pile incorporates smart technology to monitor performance and the void can be used to integrate renewable technologies. HIPER® Pile helps achieve carbon reduction and circular economy aims, with its 80% reduction in materials and emissions, greater on-site productivity and reusability.
Latent Thermal Storage
Heat physically changes a material either by melting, crystallisation or evaporation. Energy density typically 70 kWh/m3
An example of a latent heat storage system has been developed by a UK company called Sunamp. This is a compact heat battery technology, which consist of high energy density, high power density batteries which store heat generated from renewable electricity either via a heat pump or via a direct electric heater. This can replace the need for a hot water cylinder or gas-fired boiler and, if controlled by an intelligent energy management system, can optimise charging to use renewable energy when it is available. This system is well-suited to domestic buildings due to its compact nature, but is also applicable to commercial and industrial use.
Phase change materials (PCMs) can be used in elements of the building fabric, e.g. ceilings, to replicate the effect of thermal mass, to avoid overheating in summer and to increase heat retention in winter.
Another example of a latent heat storage system is the Warmstone Heat Battery developed by Caldera. This heat battery uses electrical elements that can be charged either by renewable energy sources or from the grid at off-peak, low-cost times of day. Heat can be stored in the battery until it is needed, providing both hot water and heating, using a heat exchanger. Furthermore, the battery is manufactured using a combination of recycled and natural materials, and is 100% recyclable at its end of life.
Emerging Technology: Thermochemical heat storage
Energy density typically 140-830 kWh/m3
A research group at SPECIFIC is developing a novel form of inter-seasonal heat storage, using Salt in Matrix (or “SIM”) material, which is able to store thermal energy through a thermochemical process1. This has a much higher density than water, so has the ability to store more heat in a smaller unit, and has huge potential to reduce energy use and fuel poverty.
Thermal energy (heat) is stored by passing hot air over the SIM, creating a chemical reaction that locks the energy into the material. The reaction is reversed exothermically, meaning that heat is released, by passing damp air over the SIM. Provided the SIM is kept dry it is able to store the heat indefinitely. This makes it suitable for the inter-seasonal storage of heat and for transporting heat from one location to another. As the heat is stored chemically, there is no self-discharge that is associated with other thermal storage technologies due to thermal losses. Provided the material is kept dry, the energy is stored at ambient temperature indefinitely.
It can be used in the following ways:
•Storing solar energy generated in summer months for use in winter;
•Storing waste heat from industrial/manufacturing processes and transporting this for use in housing;
•Storing heat generated by one building to heat another that needs the heat, providing an alternative to expensive and energy intensive air-conditioning, which would otherwise be needed in, for example, office buildings. By drawing the heat away from a space into a thermal store, no additional cooling is needed and the heat can be used where needed.
While reducing imported heat for buildings and hence energy costs, this also has the potential to protect the environment from rising CO2 emissions, helping the UK meet it’s Net Zero Carbon goal. Use of industrial waste heat could also ensure that it is economically viable for manufacturing companies to remain in the UK, reducing their Climate Levy charges and reducing their fuel bills, hence providing economic stability for UK businesses.
Note: This technology is not yet commercially available, but further information can be found here.