With the rapid rise of renewable energy technologies, there’s a risk of solving one environmental problem and creating another. Dr Matthew Davies is our Applied Photochemistry group lead. His research focusses on the materials and processes that will enable re-use and re-manufacture of next generation solar-PV materials.
Developing sustainable renewable energy technologies is vital in the move to decarbonise industry. Renewable energy technologies, including solar, have enormous socio-economic benefits and are significantly better for the environment than fossil-fuels, which continue to increase atmospheric greenhouse gas (GHG) levels and accelerate climate change.
The cost of solar energy technology has plummeted, with silicon PV (Si-PV) now (in some locations) cheaper than fossil fuels, resulting in a dramatic growth in its use. The average lifespan of a Si-PV module is around 25 years, but when the solar panels no longer work the material is not currently easy to recycle due to toxic chemicals: it often requires energy intensive or costly processes which degrade materials and prevent re-use in new solar panels. By 2050, there will be over 60 million tons of waste silicon panels.
Although viewed as ‘green’, there are significant environmental impacts associated with renewable technologies like photovoltaics (PV), and technologies that support renewable energy systems like batteries for energy storage. In our effort to tackle climate change quickly, it is important to make sure that renewable energy itself is also sustainable.
As well as containing hazardous chemicals, PV currently depends on the supply of critical materials. Traditionally, indium has been used in the manufacture PV modules (as well as touchscreens), but there is not enough indium on the planet to make the number of solar cells we will need to mitigate climate change.
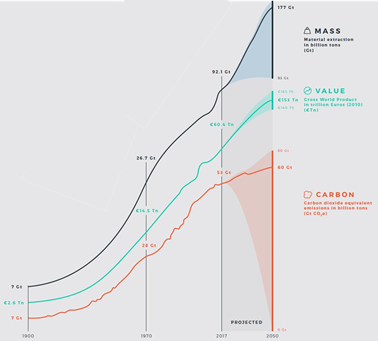
Development for material extraction (Mass), financial value creation (Value), and GHG emissions (Carbon) from 1900 to 2017 and projected to 2050. The link between the extraction of raw materials is inherently linked to the growth of value (i.e. the economy). If this continues, it will be impossible to decrease our carbon emissions and meet our climate change targets. (Circularity Gap Report 2019)
Currently, PV supplies roughly 3% of global electricity, but this already depends on high quantities of tellurium, silver, semiconductor quality quartz and smaller but important fractions of indium, zinc, gallium and tin supplies. These materials are also used in other important renewable or energy efficient technologies that are needed to combat climate change – we need to avoid a scenario where renewable technologies have to compete with each other for materials as this will limit our ability to respond to climate change.
Transition to a circular economy
Current linear economic models – in which materials are extracted, used and thrown away – limits the availability of materials and increases GHG emissions associated with producing these products.
Our Applied Photochemistry Group works with the Royal Society of Chemistry to bring together government, industry and academia to advise policymakers on mitigating problems with future wastes and enhancing the efficiency of resources in the lifecycles of emerging technologies.
Widespread deployment of solar technologies must come with transition to a ‘circular economy’, in which materials are kept in service for as long as possible at the highest value possible, eventually being cycled back into the economy. When products reach the end of their useful life, the waste hierarchy (reuse > remanufacture > recycle) must be prioritised.
For solar technologies to be truly sustainable, it is vital that full lifecycle optimisation is embedded at an early stage of development so that these technologies can be integrated within a wider global circular economy. This includes:
- minimising environmental impacts associated with production, such as low energy and waste processes
- developing end-of-life strategies and processes, such as refurbishment, remanufacturing or efficient recycling
- designing for longevity, potentially with in-situ reconditioning
- selecting low-impact materials, particularly secondary and biologically derived ones
- substituting primary & critical resources, for earth abundant, renewable or recovered resources
For Si-PV this must be done retrospectively. But, for emerging technologies like the ones we work on here at SPECIFIC, this is an opportunity to develop and design the next generation of PV technology with the circular economy in mind from the start, to deliver maximum benefit to society and a truly sustainable renewable energy technology.
Designing for end of life from the start
Here at SPECIFIC, we aim to avoid the mistakes of the past and focus on sustainability, whilst still deploying renewables quickly. We work on realistic alternatives to traditional Si-PV, and investigating the use of low-cost, printable, earth-abundant materials.
One challenge we are trying to address is the eco-design of devices so that they can be remanufactured into new devices at the end of life using as much of the original device as possible.
Halide perovskite solar cells (PSCs) is one extremely promising technology that we’re working on. Their remarkable evolution during the last few years, reaching power conversion efficiencies over 25%, makes them a strong candidate to develop a low-cost, low-embodied-energy, competitive PV technology.
We work on improving the efficiency and longevity of perovskite devices, and undertake life-cycle analyses to evaluate the benefit of using greener materials and re-manufacturing processes.
The next generation of PV presents a unique opportunity to design for maximum value and ease of remanufacture from the outset.
This will mean the use of primary materials (i.e. from the Earth) in future solar panels can be minimised, and it will mean that economic growth is not intrinsically linked to carbon emissions. The benefits of this for the environment, for the economy and society could be huge.
“Adopting a circular economy is the only way we’re going to reach our climate change targets.” – Dr Matthew Davies
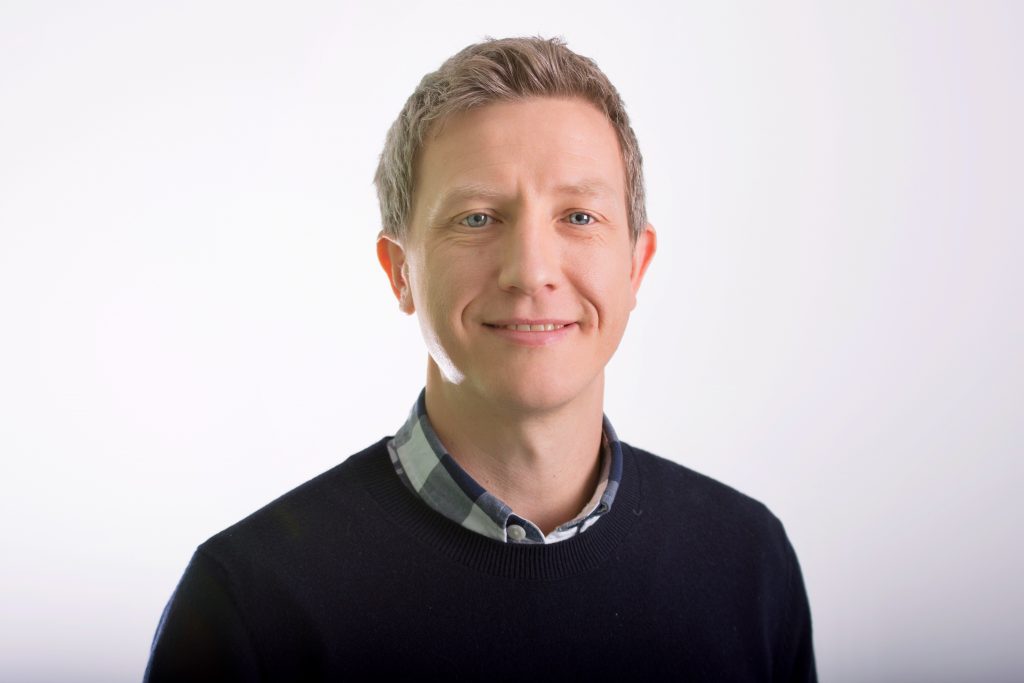
Further reading:
Mitigating Issues of Future Wastes: Enhancing Resource Productivity in Emerging Technologies
5 – Sustainable Solar Energy Collection and Storage for Rural Sub-Saharan Africa